How Light Tells Us the Story of the Universe
光如何告诉我们宇宙的故事
Author: Phil Plait 菲尔·普莱特
Length: • 7 mins
• 7 分钟
Annotated by howie.serious
由 howie.serious 注释
6 min read
Almost everything we know about the cosmos is conveyed by photons traveling across vast distances
我们对宇宙几乎所有的了解都是通过穿越广阔距离的光子传递的
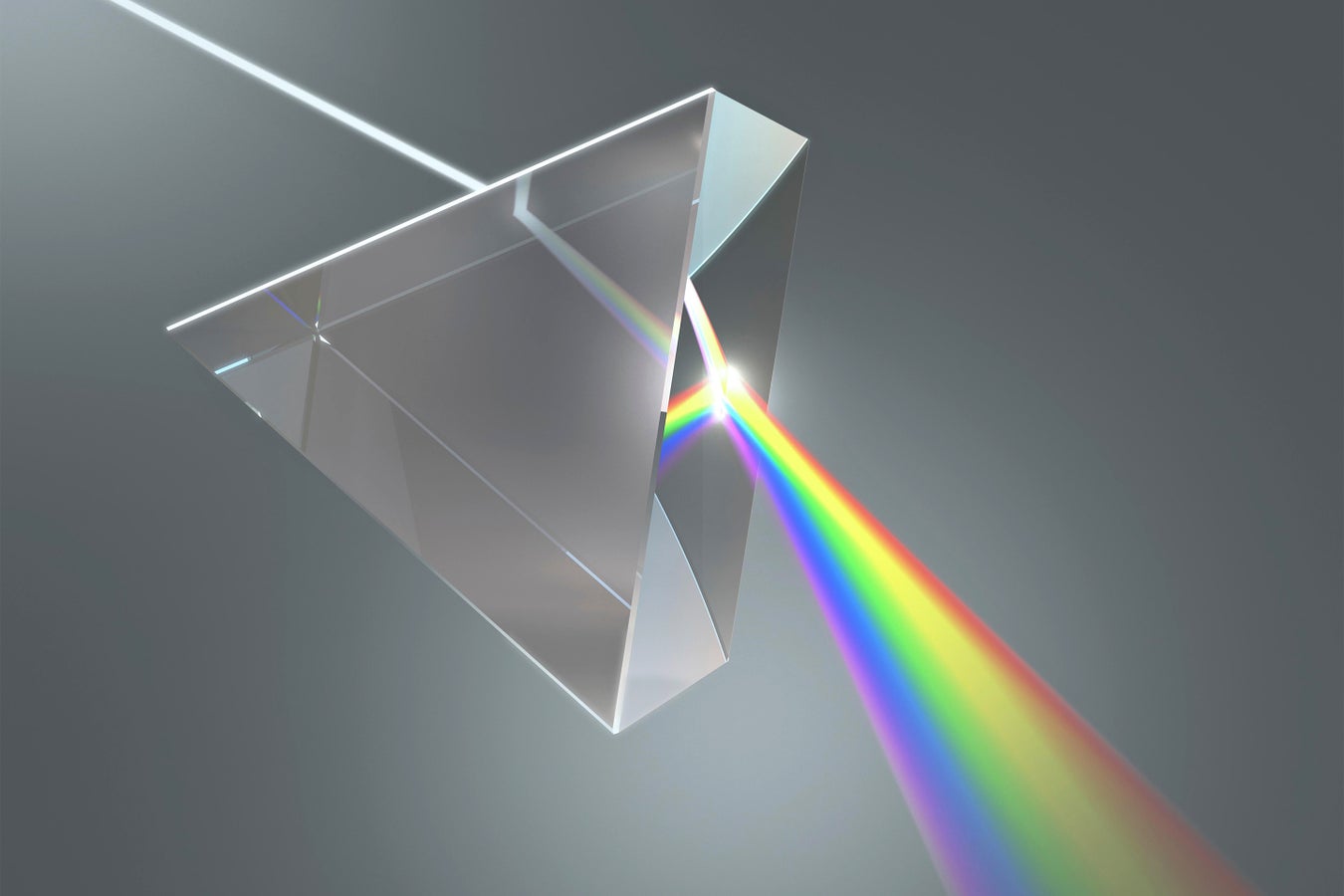
KTSDesign/科学图片库/Alamy Stock Photo
Photons are odd little beasts.
光子是奇怪的小东西。
They can act like waves. They can act like particles. They are teeny tiny messengers of force. They are carriers of energy.
它们可以表现得像波。它们可以表现得像粒子。它们是微小的力的信使。它们是能量的载体。
But most of all, they’re light. When you think of light, you’re thinking of photons. So literally, when you look around you and see, well, anything, your eyes are detecting photons that are emitted by objects like your computer screen or a lamp, photons emitted by those sources that are reflected off other objects or an absence of photons—a result of something absorbing or blocking them as they travel through space. Because of this, nearly everything we know about objects in deep space is because of light.
但最重要的是,它们是光。当你想到光时,你想到的是光子。所以,字面上,当你环顾四周看到任何东西时,你的眼睛正在检测由物体(如你的电脑屏幕或灯)发射的光子,这些光子被这些源发射出来后反射到其他物体上,或者是光子的缺失——这是由于某些东西在它们穿越空间时吸收或阻挡了它们。因此,我们对深空物体的几乎所有了解都是因为光。
Another thing photons are is weird—very, very weird.
另一个光子的特点是奇怪——非常,非常奇怪。
In many ways they behave like waves, similar to those on the beach or in your bathtub when you splash around, with crests and troughs. The distance between crests is called the wavelength, and the amplitude of a wave—its strength, effectively—is the difference in height between the trough and a crest. In a sound wave, that’s related to the volume of the sound. And in light, it’s related to the light’s intensity.
在许多方面,它们表现得像波浪,类似于海滩上的波浪或你在浴缸里戏水时的波浪,有波峰和波谷。波峰之间的距离称为波长,波的振幅——实际上是它的强度——是波谷和波峰之间的高度差。在声波中,这与声音的音量有关。而在光中,这与光的强度有关。
In other ways, photons act like subatomic particles, which can have momentum, spin, and more. It’s very difficult to wrap your head around the idea that light can be a wave and a particle, even at the same time, but quantum mechanics is exceptionally bizarre that way (which is a big reason it took so long to be accepted by scientists as a good model of reality). These properties, however, define light, and they in turn tell us a lot about the objects that emit or reflect it.
在其他方面,光子表现得像亚原子粒子,可以有动量、自旋等。很难理解光可以同时是波和粒子,即使在同一时间,但量子力学在这方面异常奇特(这也是科学家们花了很长时间才接受它作为现实的良好模型的一个重要原因)。然而,这些特性定义了光,它们反过来告诉我们很多关于发射或反射光的物体的信息。
For light, the most fundamental property is the wavelength. That describes how much energy the light has, with shorter wavelength waves having more energy than ones with longer wavelengths. More colloquially, we see this difference in wavelength (or energy) as color. When you see something as violet, for example, you’re seeing light coming from that object with a shorter wavelength. Blue has a slightly longer wavelength, green longer again, then yellow, orange and finally red, with the longest we see. We can measure the wavelengths of these colors of visible light to determine the range our eyes can detect, and it goes very roughly from 380 nanometers (nm) for violet to about 750 nm for red. (One nanometer is a billionth of a meter.)
对于光来说,最基本的特性是波长。它描述了光有多少能量,波长越短的波能量越大,波长越长的波能量越小。通俗地说,我们将这种波长(或能量)的差异视为颜色。例如,当你看到某物为紫色时,你看到的是来自该物体的短波长光。蓝色的波长稍长,绿色的更长,然后是黄色、橙色,最后是红色,红色的波长最长。我们可以测量这些可见光颜色的波长来确定我们眼睛可以检测到的范围,大约从 380 纳米(nm)的紫色到大约 750 纳米的红色。(一纳米是一米的十亿分之一。)
An aside: the frequency of light is another fundamental property and is a measure of how frequently the crests pass an observer. It’s equal to the inverse of the wavelength; in other words, the longer the wavelength, the lower the frequency, and vice versa. Scientists use both, usually picking one or the other in their calculations depending on what makes the math easier or more intuitive.
顺便说一下,光的频率是另一个基本特性,是波峰经过观察者的频率的度量。它等于波长的倒数;换句话说,波长越长,频率越低,反之亦然。科学家们使用这两者,通常根据使数学更简单或更直观的需要选择其中之一。
Our eyes have evolved cells called cones in our retinas that are sensitive to different wavelengths of light. There are three kinds: one detects a small range of wavelengths centered on red, and the others detect ranges centered on green and blue. As light hits those cells, they send signals to the brain, which combines them to create the colors we see.
我们的眼睛在视网膜中进化出了称为视锥细胞的细胞,这些细胞对不同波长的光敏感。有三种类型:一种检测以红色为中心的小范围波长,其他两种检测以绿色和蓝色为中心的波长。当光线照射到这些细胞时,它们会向大脑发送信号,大脑将这些信号结合起来,形成我们看到的颜色。
Light can have wavelengths well outside what our eyes can see, too. Scientist William Herschel figured this out in the 19th century, when he found he could still feel heat from sunlight even when it was passed through dark filters. He had discovered infrared light, with wavelengths greater than 750 nm. Beyond that, at progressively longer wavelengths, are microwaves, millimeter waves and radio waves. On the other end of the spectrum there’s ultraviolet, with wavelengths shorter than what we see as violet. Progressively shorter still are x-rays and then gamma rays, the latter with wavelengths shorter than about 10 picometers (or one one-hundredth of a nanometer).
光的波长也可以远远超出我们眼睛能看到的范围。科学家威廉·赫歇尔在 19 世纪发现了这一点,当时他发现即使阳光通过黑暗的滤光片,他仍然能感觉到热量。他发现了红外光,其波长大于 750 纳米。再往后,波长逐渐变长的是微波、毫米波和无线电波。在光谱的另一端是紫外线,其波长比我们看到的紫色还要短。再短一些的是 X 射线,然后是伽马射线,后者的波长短于约 10 皮米(或百分之一纳米)。
Because energy scales inversely with wavelength (or directly with frequency, if you prefer), gamma rays have a lot of energy, while radio waves have very little. As a category, gamma rays include any light with wavelengths less than those of x-rays (or an energy higher than x-rays). Radio waves have an open-ended range as well but at the long wavelength end of the spectrum; a wavelength measured in light-years would still be considered a radio wave.
因为能量与波长成反比(或者如果你愿意,可以说与频率成正比),伽马射线具有很高的能量,而无线电波的能量很低。作为一个类别,伽马射线包括任何波长小于 X 射线的光(或能量高于 X 射线)。无线电波在光谱的长波端也有一个开放的范围;即使波长以光年为单位测量,仍然会被认为是无线电波。
And this brings us to the universe. Excluding meteorites, which fall to the ground and can be examined, until recently all the information we got from the cosmos outside our atmosphere was from light. Stars, galaxies, dust clouds, exoplanets: all of these emit or reflect or absorb light, creating signals that travel across space for incredible distances to be detected here on Earth.
这将我们带到了宇宙。除陨石外,陨石会落到地面并可以被检查,直到最近所有我们从大气层外的宇宙中获得的信息都来自光。恒星、星系、尘云、系外行星:所有这些都发射、反射或吸收光,产生信号,穿越空间的巨大距离,在地球上被检测到。
And those signals—that light—tell us the story of the universe.
而这些信号——这些光——告诉了我们宇宙的故事。
For example, hot objects glow with their own light. They emit a wide range of colors, but at a given temperature there’s a peak wavelength where it emits the most light. That peak wavelength gets shorter as temperatures get hotter. Stars like the sun emit light most strongly in the visible spectrum. That’s no coincidence; there’s so much sunlight around that it’s a very handy tool to get information about your environment, which is why so many creatures on Earth evolved eyes to detect it. Cooler stars peak more in the red, hotter ones in the blue, and that’s why stars have colors.
例如,热物体会发出自己的光。它们发出各种颜色,但在给定温度下有一个峰值波长,它发出的光最多。随着温度升高,这个峰值波长会变短。像太阳这样的恒星在可见光谱中最强烈地发光。这并非巧合;周围有这么多阳光,这是获取环境信息的非常有用的工具,这就是为什么地球上有这么多生物进化出眼睛来检测它。较冷的恒星在红色范围内达到峰值,较热的恒星在蓝色范围内达到峰值,这就是为什么恒星有颜色的原因。
Just by measuring a star’s color you can tell its temperature. That’s an amazing thing—taking the temperature of an object that can be quintillions of kilometers away!
仅通过测量恒星的颜色,你就可以知道它的温度。这是一件了不起的事情——测量一个可能在数万亿公里之外的物体的温度!
That temperature also tells us about the physics behind the light. It takes a lot of energy to make x-rays, and even more to make gamma rays, so if we see them coming from an object, we know it packs a punch (and usually has extremely strong magnetic fields, which can supercharge subatomic particles and give them the energy needed to emit these kinds of light). Neutron stars, black holes, intergalactic gas clouds colliding, even tangled magnetic fields on the sun, can all blast out x- and gamma rays, indicating these objects’ true power.
该温度还告诉我们光背后的物理学。制造 X 射线需要大量能量,制造伽马射线需要更多能量,所以如果我们看到它们来自某个物体,我们知道它很有能量(通常具有极强的磁场,可以超充亚原子粒子并赋予它们发出这些光所需的能量)。中子星、黑洞、碰撞的星际气体云,甚至太阳上的纠缠磁场,都可以发出 X 射线和伽马射线,表明这些物体的真正力量。
For visible light (as well as ultraviolet and infrared), astronomers use filters to determine colors to measure stellar temperatures, among other things. These filters can be, for example, treated glass plates that allow a narrow range of wavelengths of light to pass through them so they can isolate colors like blue, green or red.
对于可见光(以及紫外线和红外线),天文学家使用滤光片来确定颜色以测量恒星温度等。这些滤光片可以是例如经过处理的玻璃板,允许窄范围的光波长通过它们,以便它们可以隔离蓝色、绿色或红色等颜色。
But we can do much better. Passing light through a prism or over a grating—a piece of glass or metal that has a series of very fine parallel grooves in it—breaks up light into many individual colors. The resulting spectrum, as it’s called, can have thousands of individual wavelengths detectable in it. And this ability is key to understanding the universe. And for once, I’m not exaggerating even a little bit.
但我们可以做得更好。通过棱镜或光栅——一块有一系列非常细平行槽的玻璃或金属——通过光线可以将光分解成许多单独的颜色。结果光谱(如其所称)可以检测到其中的数千个单独波长。这种能力是理解宇宙的关键。这一次,我一点也没有夸张。
Different atomic elements absorb and emit light at very specific colors (the electrons zipping around the atom’s nucleus can only absorb discrete amounts of energy, a fact that gave rise to the development of quantum mechanics in the first place). Hydrogen, for example, strongly absorbs light at 656 nm (in the red). Some stellar spectra show that as a deep dark band or line at that wavelength in the light they emit. This is how astronomers first determined stars are mostly made of hydrogen. But often we see absorption lines for many different elements at different wavelengths, allowing us to determine more thoroughly what stars are made of; this technique can also tell us what elements are in gas clouds and even the atmospheres of exoplanets orbiting other stars.
不同的原子元素在非常特定的颜色下吸收和发射光(围绕原子核旋转的电子只能吸收离散的能量,这一事实最初促成了量子力学的发展)。例如,氢在 656 纳米(红色)处强烈吸收光。一些恒星光谱显示在它们发射的光中,这个波长处有一个深暗的带或线。这就是天文学家首次确定恒星主要由氢组成的方式。但我们经常在不同波长处看到许多不同元素的吸收线,这使我们能够更彻底地确定恒星的成分;这种技术还可以告诉我们气体云和甚至围绕其他恒星运行的系外行星的大气中有哪些元素。
And there’s so much more. If an object is moving toward you, the wavelengths of the light it emits get compressed, similar to how sound waves from an approaching ambulance’s siren will be compressed and rise in pitch. For light, we call this a blueshift. If an object is moving away, the wavelengths of its light instead get stretched, called redshifting. Stars and galaxies rotate, shifting their light in a way that allows astronomers to determine how rapidly they’re spinning. Moreover, in the early 20th century astronomers figured out that more distant galaxies have larger redshifts, meaning the universe is expanding. By precisely measuring these redshifts they could also figure out how rapidly the cosmos is expanding as well—and how long it’s been doing so, giving us its age of 13.8 billion years.
还有更多。如果一个物体向你移动,它发射的光的波长会被压缩,类似于接近的救护车的警笛声波会被压缩并升高音调。对于光,我们称之为蓝移。如果一个物体远离你,它的光的波长会被拉伸,称为红移。恒星和星系旋转,使它们的光发生变化,从而使天文学家能够确定它们的旋转速度。此外,在 20 世纪初,天文学家发现更远的星系有更大的红移,这意味着宇宙在膨胀。通过精确测量这些红移,他们还可以确定宇宙膨胀的速度——以及它膨胀了多长时间,从而得出宇宙的年龄为 138 亿年。
I mentioned light was the only way we would get information from space until recently. Now we can also detect other sorts of cosmic messengers: subatomic particles such as neutrinos and cosmic rays and other phenomena such as gravitational waves, literally ripples in the fabric of spacetime. We can combine that information with the light we detect, meaning we’re now in the era of “multimessenger” astronomy, a powerful tool to help us understand what’s going on over our head.
我提到过光是我们从太空获取信息的唯一方式直到最近。现在我们还可以检测到其他类型的宇宙信使:如中微子和宇宙射线等亚原子粒子以及引力波等现象,字面上是时空结构中的涟漪。我们可以将这些信息与我们检测到的光结合起来,这意味着我们现在处于“多信使”天文学时代,这是一种强大的工具,帮助我们理解头顶上发生的事情。
So remember all this when you go outside on a clear night. The light you see from those stars twinkling above you traveled hundreds of trillions of kilometers at a ridiculous speed for years or decades, passing through space and Earth’s atmosphere and then finally being stopped by and absorbed by the cells in your eye. Light is a way for the universe to literally touch you. The least we can do is use that light to learn as much as we can about it.
所以,当你在晴朗的夜晚走到外面时,请记住这一切。你看到的那些在你头顶闪烁的星星发出的光,以惊人的速度穿越了数百兆公里,经过了数年或数十年的时间,穿过了太空和地球的大气层,最终被你的眼细胞吸收。光是宇宙触碰你的方式。我们至少可以利用这些光尽可能多地了解宇宙。
Phil Plait is a professional astronomer and science communicator in Virginia. He writes the Bad Astronomy Newsletter. Follow him online.
菲尔·普莱特是弗吉尼亚州的一名专业天文学家和科学传播者。他撰写了坏天文学通讯。在网上关注他。